It took me a few minutes to find the butterflies (visual pattern recognition is not one of my strengths) but here they are,
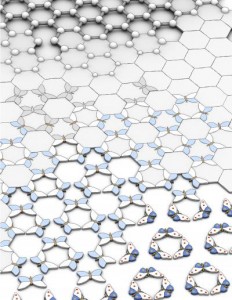
Caption: Graphene, combined with white graphene, forms stunning ‘butterfly’ images.
Credit: The University of Manchester
The May 15, 2013 University of Manchester news release (on EurekAlert and on the University of Manchester news site) describes how the ‘butterflies’ are formed,
Writing in Nature, a large international team led Dr Roman Gorbachev from The University of Manchester shows that, when graphene placed on top of insulating boron nitride, or ‘white graphene’, the electronic properties of graphene change dramatically revealing a pattern resembling a butterfly.
The pattern is referred to as the elusive Hofstadter butterfly that has been known in theory for many decades but never before observed in experiments.
More of the science needs to be explained before moving on with the ‘butterflies’ (from the news release),
One of the most remarkable properties of graphene is its high conductivity – thousands of times higher than copper. This is due to a very special pattern created by electrons that carry electricity in graphene. The carriers are called Dirac fermions and mimic massless relativistic particles called neutrinos, studies of which usually require huge facilities such as at CERN. The possibility to address similar physics in a desk-top experiment is one of the most renowned features of graphene.
Now the Manchester scientists have found a way to create multiple clones of Dirac fermions. Graphene is placed on top of boron nitride so that graphene’s electrons can ‘feel’ individual boron and nitrogen atoms. Moving along this atomic ‘washboard’, electrons rearrange themselves once again producing multiple copies of the original Dirac fermions.
Here’s where the butterflies appear (from the news release),
The researchers can create even more clones by applying a magnetic field. The clones produce an intricate pattern; the Hofstadter butterfly. It was first predicted by mathematician Douglas Hofstadter in 1976 and, despite many dedicated experimental efforts, no more than a blurred glimpse was reported before.
In addition to the described fundamental interest, the Manchester study proves that it is possible to modify properties of atomically-thin materials by placing them on top of each other. This can be useful, for example, for graphene applications such as ultra-fast photodetectors and transistors, providing a way to tweak its incredible properties.
Coincidentally, another team has also observed the Hofstadter butterfly on a graphene substrate. From the May 16, 2013 news item on Azonano,
Two research teams at the National High Magnetic Field Laboratory (MagLab) broke through a nearly 40-year barrier recently when they observed a never-before-seen energy pattern.
…
“The observation of the ‘Hofstadter butterfly’ marks a real landmark in condensed matter physics and high magnetic field research,” said Greg Boebinger, director of the MagLab. “It opens a new experimental direction in materials research.”
This groundbreaking research demanded the ability to measure samples of materials at very low temperatures and very high magnetic fields, up to 35 tesla. Both of those conditions are available at the MagLab, making it an international destination for scientific exploration.
The unique periodic structure used to observe the butterfly pattern was composed of boron nitride (BN) and graphene.
The May 15, 2013 Florida State University news release by Kristin Roberts, which originated the news item, describes the two teams using the MagLab facilities for their ‘butterfly’ observations,
One research team was led by Columbia University’s Philip Kim and included researchers from City University of New York, the University of Central Florida, Tohoku University and the National Institute for Materials Science in Japan. The team’s work will be published today in the Advanced Online Publication of the journal Nature. Similar results were discovered at the MagLab by a group led by Pablo Jarillo-Herrero and Raymond Ashoori at MIT, as well as scientists from Tohoku University and the National Institute for Materials Science in Japan. Their work is expected to be published soon.
For those who just can’t get enough graphene butterflies here are citations for and links to both recently published papers (the Jarillo-Herrero/Ashoori team will be publishing their work soon).
Cloning of Dirac fermions in graphene superlattices by L. A. Ponomarenko, R. V. Gorbachev, G. L. Yu,D. C. Elias, R. Jalil, A. A. Patel, A. Mishchenko, A. S. Mayorov, C. R. Woods, J. R. Wallbank, M. Mucha-Kruczynski, B. A. Piot, M. Potemski, I. V. Grigorieva, K. S. Novoselov, F. Guinea, V. I. Fal’ko & A. K. Geim. Nature doi:10.1038/nature12187 Published online
and,
Hofstadter’s butterfly and the fractal quantum Hall effect in moiré superlattices by C. R. Dean, L. Wang, P. Maher, C. Forsythe, F. Ghahari, Y. Gao, J. Katoch, M. Ishigami, P. Moon, M. Koshino, T. Taniguchi, K. Watanabe, K. L. Shepard, J. Hone & P. Kim. Nature (2013) doi:10.1038/nature12186 Published online 15 May 2013
Both papers are behind paywalls.