A September 8, 2021 news item on phys.org announces research that could make the process of whitening teeth safer,
Most people would like to flash a smile of pearly whites, but over time teeth can become stained by foods, beverages and some medications. Unfortunately, the high levels of hydrogen peroxide in dentists’ bleaching treatments can damage enamel and cause tooth sensitivity and gum irritation. Now, researchers reporting in ACS Applied Materials & Interfaces have developed a gel that, when exposed to near infrared (NIR) light, safely whitens teeth without the burn.
…
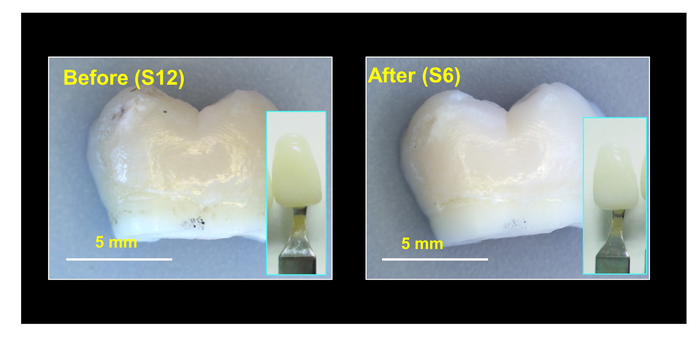
A September 8, 2021Amercian Chemical Society (ACS) news release (also on EurekAlert), which originated the news item, provides more detail,
The growing demand for selfie-ready smiles has made tooth whitening one of the most popular dental procedures. Treatments at a dentist’s office are effective, but they use high-concentration hydrogen peroxide (30–40%). Home bleaching products contain less peroxide (6–12%), but they usually require weeks of treatment and don’t work as well. When a bleaching gel is applied to teeth, hydrogen peroxide and peroxide-derived reactive oxygen species (mainly the hydroxyl radical) degrade pigments in stains. The hydroxyl radical is much better at doing this than hydrogen peroxide itself, so researchers have tried to improve the bleaching capacity of low-concentration hydrogen peroxide by boosting the generation of powerful hydroxyl radicals. Because previous approaches have had limitations, Xingyu Hu, Li Xie, Weidong Tian and colleagues wanted to develop a safe, effective whitening gel containing a catalyst that, when exposed to NIR light, would convert low levels of hydrogen peroxide into abundant hydroxyl radicals.
The researchers made oxygen-deficient titania nanoparticles that catalyzed hydroxyl radical production from hydrogen peroxide. Exposing the nanoparticles to NIR light increased their catalytic activity, allowing them to completely bleach tooth samples stained with orange dye, tea or red dye within 2 hours. Then, the researchers made a gel containing the nanoparticles, a carbomer gel and 12% hydrogen peroxide. They applied it to naturally stained tooth samples and treated them with NIR light for an hour. The gel bleached teeth just as well as a popular tooth whitening gel containing 40% hydrogen peroxide, with less damage to enamel. The nanoparticle system is highly promising for tooth bleaching and could also be extended to other biomedical applications, such as developing antibacterial materials, the researchers say.
The authors acknowledge funding from the National Natural Science Foundation of China, the National Key R&D Program of China and the Key Technologies R&D Program of Sichuan Province.
…
Here’s a link to and a citation for the paper,
Photothermal-Enhanced Fenton-like Catalytic Activity of Oxygen-Deficient Nanotitania for Efficient and Safe Tooth Whitening by Xingyu Hu, Li Xie, Zhaoyu Xu, Suru Liu, Xinzhi Tan, Ruojing Qian, Ruitao Zhang, Mingyan Jiang, Wenjia Xie, and Weidong Tian. ACS Appl. Mater. Interfaces 2021, 13, 30, 35315–35327 Publication Date: July 22, 2021 DOI: https://doi.org/10.1021/acsami.1c06774 Copyright © 2021 American Chemical Society
This paper is behind a paywall.