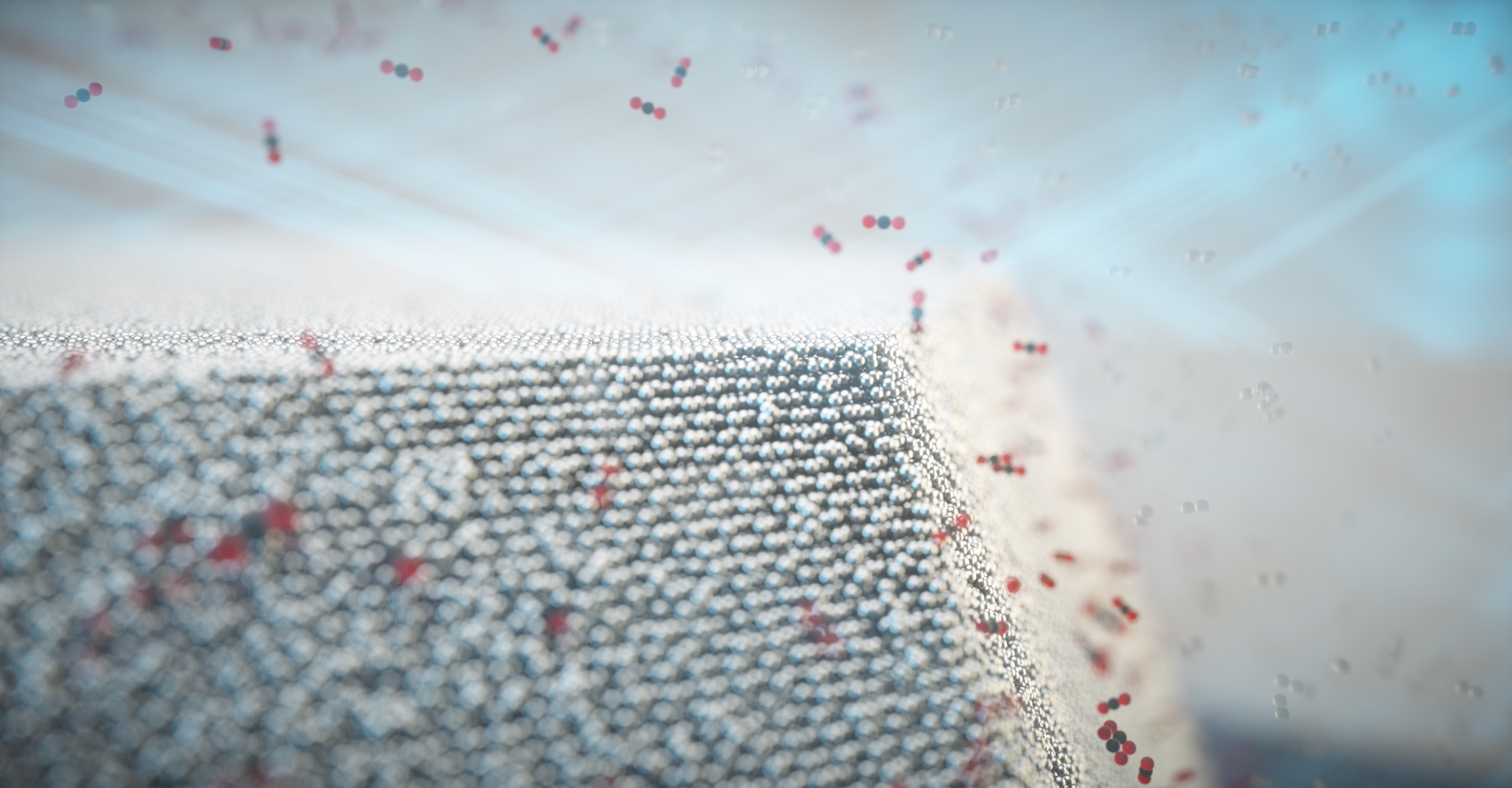
An October 1, 2021 news item on ScienceDaily announces research enabling scientists to observe a single nanoparticle at work,
A DESY-led research team has been using high-intensity X-rays to observe a single catalyst nanoparticle at work. The experiment has revealed for the first time how the chemical composition of the surface of an individual nanoparticle changes under reaction conditions, making it more active. The team led by DESY’s Andreas Stierle is presenting its findings in the journal Science Advances. This study marks an important step towards a better understanding of real, industrial catalytic materials.
An October 1, 2021 Deutsches Elektronen-Synchrotron (DESY) press release (also on EurekAlert), which originated the news item, explains why this research is important and provides more technical details,
Catalysts are materials that promote chemical reactions without being consumed themselves. Today, catalysts are used in numerous industrial processes, from fertiliser production to manufacturing plastics. Because of this, catalysts are of huge economic importance. A very well-known example is the catalytic converter installed in the exhaust systems of cars. These contain precious metals such as platinum, rhodium and palladium, which allow highly toxic carbon monoxide (CO) to be converted into carbon dioxide (CO2) and reduce the amount of harmful nitrogen oxides (NOx).
“In spite of their widespread use and great importance, we are still ignorant of many important details of just how the various catalysts work,” explains Stierle, head of the DESY NanoLab. “That’s why we have long wanted to study real catalysts while in operation.” This is not easy, because in order to make the active surface as large as possible, catalysts are typically used in the form of tiny nanoparticles, and the changes that affect their activity occur on their surface.
Surface strain relates to chemical composition
In the framework of the EU project Nanoscience Foundries and Fine Analysis (NFFA), the team from DESY NanoLab has developed a technique for labelling individual nanoparticles and thereby identifying them in a sample. “For the study, we grew nanoparticles of a platinum-rhodium alloy on a substrate in the lab and labelled one specific particle,” says co-author Thomas Keller from DESY NanoLab and in charge of the project at DESY. “The diameter of the labelled particle is around 100 nanometres, and it is similar to the particles used in a car’s catalytic converter.” A nanometre is a millionth of a millimetre.
Using X-rays from the European Synchrotron Radiation Facility ESRF in Grenoble, France, the team was not only able to create a detailed image of the nanoparticle; it also measured the mechanical strain within its surface. “The surface strain is related to the surface composition, in particular the ratio of platinum to rhodium atoms,” explains co-author Philipp Plessow from the Karlsruhe Institute of Technology (KIT), whose group computed strain as a function of surface composition. By comparing the observed and computed facet-dependent strain, conclusions can be drawn concerning the chemical composition at the particle surface. The different surfaces of a nanoparticle are called facets, just like the facets of a cut gemstone.
When the nanoparticle is grown, its surface consists mainly of platinum atoms, as this configuration is energetically favoured. However, the scientists studied the shape of the particle and its surface strain under different conditions, including the operating conditions of an automotive catalytic converter. To do this, they heated the particle to around 430 degrees Celsius and allowed carbon monoxide and oxygen molecules to pass over it. “Under these reaction conditions, the rhodium inside the particle becomes mobile and migrates to the surface because it interacts more strongly with oxygen than the platinum,” explains Plessow. This is also predicted by theory.
“As a result, the surface strain and the shape of the particle change,” reports co-author Ivan Vartaniants, from DESY, whose team converted the X-ray diffraction data into three-dimensional spatial images. “A facet-dependent rhodium enrichment takes place, whereby additional corners and edges are formed.” The chemical composition of the surface, and the shape and size of the particles have a significant effect on their function and efficiency. However, scientists are only just beginning to understand exactly how these are connected and how to control the structure and composition of the nanoparticles. The X-rays allow researchers to detect changes of as little as 0.1 in a thousand in the strain, which in this experiment corresponds to a precision of about 0.0003 nanometres (0.3 picometres).
Crucial step towards analysing industrial catalyst materials
“We can now, for the first time, observe the details of the structural changes in such catalyst nanoparticles while in operation,” says Stierle, Lead Scientist at DESY and professor for nanoscience at the University of Hamburg. “This is a major step forward and is helping us to understand an entire class of reactions that make use of alloy nanoparticles.” Scientists at KIT and DESY now want to explore this systematically at the new Collaborative Research Centre 1441, funded by the German Research Foundation (DFG) and entitled “Tracking the Active Sites in Heterogeneous Catalysis for Emission Control (TrackAct)”.
“Our investigation is an important step towards analysing industrial catalytic materials,” Stierle points out. Until now, scientists have had to grow model systems in the laboratory in order to conduct such investigations. “In this study, we have gone to the limit of what can be done. With DESY’s planned X-ray microscope PETRA IV, we will be able to look at ten times smaller individual particles in real catalysts, and under reaction conditions.”
DESY is one of the world’s leading particle accelerator centres and investigates the structure and function of matter – from the interaction of tiny elementary particles and the behaviour of novel nanomaterials and vital biomolecules to the great mysteries of the universe. The particle accelerators and detectors that DESY develops and builds at its locations in Hamburg and Zeuthen are unique research tools. They generate the most intense X-ray radiation in the world, accelerate particles to record energies and open up new windows onto the universe. DESY is a member of the Helmholtz Association, Germany’s largest scientific association, and receives its funding from the German Federal Ministry of Education and Research (BMBF) (90 per cent) and the German federal states of Hamburg and Brandenburg (10 per cent).
Here’s a link to and a citation for the paper,
Single alloy nanoparticle x-ray imaging during a catalytic reaction by Young Yong Kim, Thomas F. Keller, Tiago J. Goncalves, Manuel Abuin, Henning Runge, Luca Gelisio, Jerome Carnis, Vedran Vonk, Philipp N. Plessow, Ivan A. Vartaniants, Andreas Stierle. Science Advances • 1 Oct 2021 • Vol 7, Issue 40 • DOI: 10.1126/sciadv.abh0757
This paper is open access.